AST (Antimicrobial susceptibility testing ) system on a microfluidic device capable of delivering results in under 6 hours was developed in the Levenberg’s lab. Using an automated algorithm for data analysis, the system is capable of determining whether bacteria from an infection site is resistant or susceptible to the tested antibiotic. This technology was licensed to Nanosynex, and now-a-days, Nanosynex takes this proof of concept from the lab to the market.
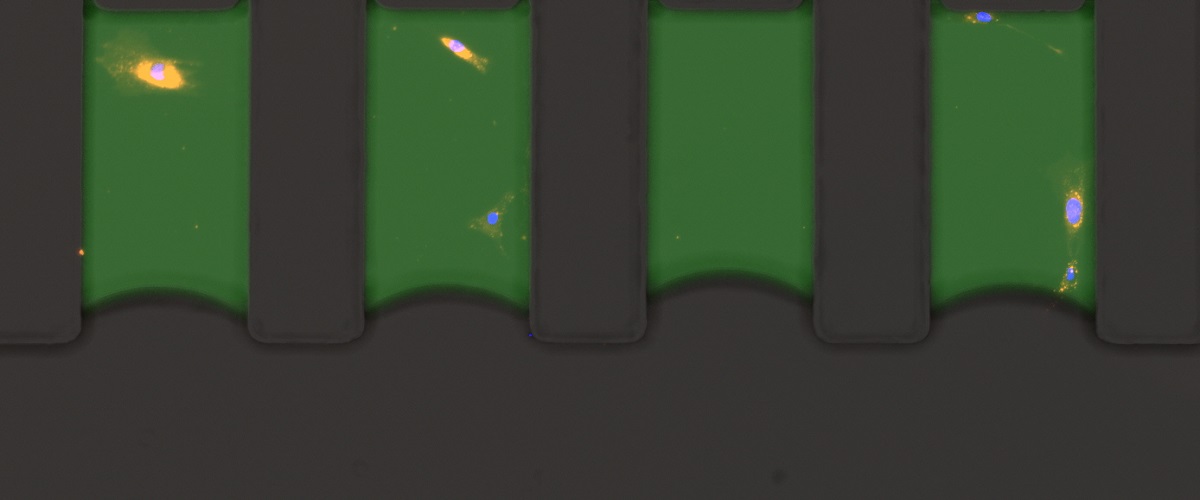